Lysine-modulated synthesis of enzyme-embedded hydrogen-bonded organic frameworks for efficient carbon dioxide fixation
Abstract
Carbonic anhydrase (CA) is an important carbon fixation enzyme. Immobilization of CA can expand its application in the realm of adsorption, catalysis, and so on. As a typical metal-free framework, hydrogen-bonded organic frameworks (HOFs) featuring mild synthesis process, exquisite framework structure and good enzyme compatibility have been used for enzyme embedding. However, the catalytic performance of CA-embedded HOFs (CA@HOFs) is limited by the micropore size of HOFs and the slow adsorption of CO2. Herein, CA@Lys-HOF-1 was synthesized by introducing lysine (Lys), a basic amino acid, during the coprecipitation of CA and HOFs for CO2 fixation. The addition of Lys enlarged the average pore size of HOF-1 from 1.8 to 3.2 nm, whereas the introduced -NH2 groups increased the initial adsorption of CO2 from 0.55 to 1.21 cm3 g-1. Compared to CA@HOF-1, the activity of CA@Lys-HOF-1 was enhanced by 71.25%, and the corresponding production of CaCO3 was enhanced by 12.7%. After eight reaction cycles, CA@Lys-HOF-1 still maintained an output of 9.97 mg of CaCO3 every 5 min, 83.7% of the initial production. It is hoped that the CA@Lys-HOF-1 reported offers a platform for efficient and continuous fixation of CO2.
Keywords
INTRODUCTION
Carbon dioxide (CO2) capture and utilization (CCU) is one of the ever-increasing research topics which can contribute to addressing environmental and ecological issues[1-4]. Enzymes such as formate dehydrogenase (FDH), ribulose-1, 5-bisphosphate carboxylase/oxygenase (RubisCO), and carbonic anhydrase (CA) can accurately activate CO2 to lower the reaction energy barrier, which have received great attention as a green and feasible solution to CCU[5-7]. CA exhibits the highest catalytic rate among all carbon-fixation enzymes, and thus has good potential for the selective transformation of CO2 to HCO3-[8]. However, enzymes that leave the bodies of organisms are easy to inactivate and difficult to reuse[9-11]. One frequently used method to address the above issues is to immobilize enzymes inside carrier materials[12].
Porous framework materials, such as metal-organic frameworks (MOFs)[13-17], covalent organic frameworks (COFs)[18-22], and hydrogen-bonded organic frameworks (HOFs)[23-25], bearing high specific surface area, high porosity, exquisite framework structure and excellent designability, are emerging carriers for enzyme immobilization. Particularly, HOFs are framework materials linked by hydrogen bonds[26-29], which can be reversibly repaired by simple recrystallization[30,31] and possess better biocompatibility due to the absence of metal ions[32,33]. These advantages make HOFs an excellent candidate as enzyme immobilization carriers[34]. For example, HOF-21 synthesized by Bao et al. can recover its original structure after immersion in aqueous or anionic source solution for 48 h[35]. Tang et al. designed TA-HOFs capable of in situ embedding enzymes with different surface charges and molecular weights[36]. Enzyme in TA-HOFs exhibited remarkably enhanced stability. However, HOFs with micropore size and balanced -NH2/-COOH groups usually showed restricted mass transfer and lower affinity with CO2, therefore exhibiting reduced apparent enzyme activity.
Herein, HOF-1 composed of two units of Tetrakis(4-amidiniumphenyl)methane and tetrakis
EXPERIMENTAL
Materials and chemicals
Tetrakis(4-amidiniumphenyl)methane tetrahydrochloride (TAM, 95%) was purchased from Jilin Chinese Academy of Sciences-Yanshen Technology Co., Ltd. Tetrakis(4-carboxyphenyl)methane (C29H20O8, 98%) was obtained from Shanghai Macklin Biochemical Technology Co., Ltd. γ-poly-l-glutamic acid (PLGA, 92%), glycine (C2H5NO2, 98%), serine (C3H7NO3, 98%), arginine (C6H14N4O2, 98%), and lysine (C6H14N2O2, 98%) were obtained from Shanghai Aladdin Bio-Chem Technology Co., Ltd. Carbonic anhydrase
Synthesis of HOF-1
Tetrakis(4-amidiniumphenyl)methane tetrahydrochloride (10 mg) was dissolved in H2O (2.5 mL) to form solution A. Tetrakis(4-carboxyphenyl)methane (7.5 mg) was dispersed in H2O (2375 μL), followed by the addition of aqueous ammonium hydroxide solution (1% v/v, 125 μL) to form solution B. Thereafter, solution B was added to solution A under stirring conditions at room temperature. Precipitation occurred immediately upon mixing. The reaction mixture was left to stir gently in the dark for 1 h. The HOF-1 material was then recovered by centrifugation, washed, dispersed, and centrifuged three times in H2O to remove any unreacted precursors.
Synthesis of CA@HOF-1
Tetrakis(4-amidiniumphenyl)methane tetrahydrochloride (10 mg) was dissolved in H2O (1.25 mL) to form solution A. An aqueous solution of CA (1.25 mL of 2 mg mL-1 stock solution) was added to solution A and stirred at room temperature for 10 min to form solution B. Tetrakis(4-carboxyphenyl)methane (7.5 mg) was dissolved in 2375 μL of H2O and 125 μL of 1% NH4OH to form solution C. Solution C was then added dropwise to solution B under stirring. The mixture was then left to stir gently for another 1 h to ensure the completion of the synthesis. Thereafter, CA@HOF-1 was collected by centrifugation and then washed, dispersed, and centrifuged three times in H2O to remove the unreacted precursors and loosely adsorbed CA.
Synthesis of CA@amino acid-HOF-1
Tetrakis (4-carboxyphenyl) methane (7.5 mg) was dissolved in 2375 μL of H2O and 125 μL of 1% NH4OH to form solution A. An aqueous solution of CA (0.625 mL of 4 mg mL-1 stock solution) and amino acid
Characterization
The sample morphologies were analyzed by scanning electron microscopy (SEM, S-4800, Hitachi) and transmission electron microscopy (TEM, JEM-100CX II, JEOL). The chemical compositions of the samples were identified by Fourier transform infrared (FTIR) spectrometer (Nicolet-6700, Nicolet). The crystal structures of the samples were identified by X-ray diffraction (XRD, X' Pert Pro), with 2-theta ranging from 10° to 90° by a step width of 0.033° with 15.24° min-1 speed at 40 mA and 40 kV. The specific surface area and pore size distribution of the samples were examined by Brunauer-Emmett-Teller (BET) method based on N2 adsorption/desorption isotherms on an AUTOSORB-1 surface area and pore size analyzer (Quantachrome Instruments).
Conversion of CO2
CA@HOF-1 and CA@Lys-HOF-1 were used for the conversion of CO2. In a typical experiment, nitrogen was first injected into the aqueous phase of the reaction device for 10 min to remove CO2 gas in the solution. Then, CO2 with a rate of 25 mL min-1 was injected into the system. A sample was taken every 5 min for follow-up reaction. Specifically, pH value of the reaction solution was detected by a pH meter and kept at 7.9, which was adjusted by adding 5 mol L-1 NaOH. Then, a certain amount of reaction solution was mixed with 670 mmol L-1 calcium chloride solution. The mixture was shaken at 200 r min-1 to form CaCO3 precipitate. The precipitated CaCO3 was filtered by a filter paper with an average pore diameter of 2.5 μm, which was then dried overnight and weighed to determine the relative yield of CaCO3.
RESULTS AND DISCUSSION
Preparation and Characterization of CA@HOF-1 and CA@Lys-HOF-1
A reported HOF material (HOF-1) was chosen for the embedding of CA[37]. The preparation and structure regulation of CA@HOF-1 are shown in Figure 1. Briefly, HOF-1 was prepared by mixing tetrakis
The topological structures of all samples were also examined by SEM and TEM. As shown in Figure 2 and Supplementary Figure 4, both CA@HOF-1 and CA@Lys-HOF-1 maintain the rod-like structure of HOF-1 with similar dimensions (around 300 nm wide). This indicates that the incorporation of CA and Lys did not alter the structure and size of HOF-1 during crystallization. As depicted in Figure 2C, some defects can be observed on the surface of CA@Lys-HOF-1, while CA@HOF-1 and HOF-1 remain intact. Figure 2D further shows that CA@HOF-1 and CA@Lys-HOF-1 well maintain the crystal structure of HOF-1 with the characteristic peaks at 2θ values of 8.6°, and 17.4°. The results of FTIR analysis of CA@HOF-1 and CA@Lys-HOF-1 are shown in Figure 2E. The absorption band centered at around 1030 cm-1 may be assigned to the N-H bending vibration of amide in Lys, indicating the introduction of Lys into HOF-1. To reveal the variation of surface area and pore size distribution of HOF-1 after modulation and enzyme embedding, the N2 adsorption-desorption isotherms of HOF-1, CA@HOF-1, and CA@Lys-HOF-1 were examined
Figure 2. TEM images of (A) HOF-1; (B) CA@HOF-1; and (C) CA@Lys-HOF-1; (D) XRD patterns; (E) FTIR spectra; (F) pore size distribution; and (G) CO2 adsorption capacity of HOF-1, CA@HOF-1, and CA@Lys-HOF-1.
BET analysis and initial CO2 adsorption volume of HOF-1, CA@HOF-1, and CA@Lys-HOF-1
HOF-1 | CA@HOF-1 | CA-Lys@HOF-1 | |
Surface area (m2 g-1) | 13.1 | 14.9 | 19.7 |
Main pore diameter (nm) | 1.8 | 3.0 | 3.2 |
Initial CO2 adsorption volume (cm3 g-1) | -0.025 | 0.546 | 1.214 |
Subsequently, EDS mapping was performed to further investigate whether enzymes were embedded in HOF-1. As shown in Figure 3, the distribution of C and N (the main elements in the two monomers of HOF-1) match the morphology of materials, which can also prove the successful synthesis of HOF-1. Particularly, S (the characteristic element of CA) is uniformly dispersed in the two samples of CA@HOF-1 and CA@Lys-HOF-1, indicating the successful embedding of CA.
Catalytic activity of CA@Lys-HOF-1
In this work, five different types of (poly) amino acids [Supplementary Figure 7] were adopted to regulate the structure and catalytic performance of CA@HOF-1. Specifically, the hydrogen bonds in HOF-1 are formed between -NH2 and -COOH of the two monomers, while amino acids are organic compounds containing both -NH2 and -COOH in the molecule. Amino acids may occupy part of the hydrogen bond formation site, and interfere with the synthesis of HOF-1 through competitive ligand interaction, thus altering the pore size of HOF-1 and influencing the mass transfer.
When γ-poly-l-glutamic acid (PLGA) was introduced, its -COOH groups can rapidly bind to monomer 1, inhibiting the formation of HOF-1. [Supplementary Figure 8]. This was probably due to the preferential combination of -COOH in PLGA and -NH2 in monomer. Among the other four types of amino acid-modulated CA@HOF-1, the CA@Lys-HOF-1 exerted the highest activity [Supplementary Figures 9 and 10].
The catalytic activity of HOF-1, Lys-HOF-1, CA@HOF-1, and CA@Lys-HOF-1 were tested by dispersing them individually in solution for CO2 conversion. The activity was reflected by detecting the pH change in the solution after the ventilation of CO2. The solution without catalyst samples was chosen as control, the ΔpH was measured in real-time, and the value at the highest point was chosen to reflect the catalyst activity. Figure 4A and B shows that the activity of CA@Lys-HOF-1 was enhanced. The higher activity of CA@Lys-HOF-1 may be attributed to the defects resulting from Lys, which promoted mass transfer. The -NH2 groups in Lys were also introduced into the material, which fortified the affinity between the materials and CO2 [Supplementary Figure 11]. Furthermore, we investigated the effect of Lys concentrations on the activity. As seen in Figure 4C and D, moderate concentration of Lys is favorable for HOF-1 modulation, thus acquiring the most active CA@Lys-HOF-1. It should be noted that the results of Arg modulation are shown in Supplementary Figure 12, which also shows the same result.
Stability and CO2 fixation ability of CA@Lys-HOF-1
Subsequently, the stability, reusability, and CO2 fixation ability of CA@Lys-HOF-1 were investigated. As shown in Figure 5, Lys-HOF-1 exhibits better protection of CA at different pH values. Regarding the thermal stability, the protective ability of Lys-HOF-1 for CA began to decrease when the temperature was higher than 60 °C. This may be owing to the weakened strength of hydrogen bonds within the carrier material after Lys modification, which made Lys-HOF-1 more prone to decomposition at higher temperatures. The reusability of CA@Lys-HOF-1 was also evaluated for its importance in industrial applications. As shown in Figure 5C, CA@Lys-HOF-1 shows excellent recyclability. After the 8th cycle of reaction, CA@Lys-HOF-1 still maintained 83.7% of its initial activity, and demonstrated unaltered morphological and crystal structure [Supplementary Figure 13].
Figure 5. (A) pH stability; (B) thermal stability; (C) reusability; and (D) production of CaCO3 enabled by CA@Lys-HOF-1.
Finally, to examine the CO2 fixation ability of CA@Lys-HOF-1, CO2 was introduced at a flow rate of 25 mL min–1, and the CaCO3 mineralization reaction was performed after 5 min of ventilation. In detail, the reaction rates of the four samples, including HOF-1, Lys-HOF-1, CA@HOF-1, and CA@ Lys-HOF-1, were assessed by measuring the amount of CaCO3 precipitate produced. As shown in Figure 5D, the CaCO3 precipitate amount of HOF-1, Lys-HOF-1, CA@HOF-1, and CA@Lys-HOF-1 is, respectively, 4.81 mg, 6.29 mg, 10.88 mg, 12.26 mg. The production of CaCO3 enabled by CA@Lys-HOF-1 was the highest, again validating the superiority of CA@Lys-HOF-1 in fortifying CO2 fixation processes. Moreover, a hot filtration[38] experiment was performed over CA@Lys-HOF-1 for CO2 mineralization. As shown in Supplementary Figure 14, no more increment in the production of CaCO3 is observed after the filtration process, suggesting the heterogeneous nature of our catalytic system.
CONCLUSIONS
In summary, HOF-1 modulated by amino acids was synthesized through a coprecipitation method for CA immobilization. By regulating the type and concentration of introduced amino acids, CA@Lys-HOF-1 with optimized activity and desirable stability was obtained. Compared with unmodulated CA@HOF-1, the activity of CA@Lys-HOF-1 was enhanced by 71.3%, whereas the CO2 fixation efficiency reflected by CaCO3 production was enhanced by 12.7%. This could be ascribed to the large pore size of CA@Lys-HOF-1 that facilitated CO2 transfer as well as the abundant surface -NH2 groups that promoted CO2 adsorption. Moreover, CA@Lys-HOF-1 maintained over 80% of the initial activity after the 8th cycle reaction. Our findings may pave the way for the immobilization of CA and other CO2-fixation enzymes.
DECLARATIONS
Authors’ contributions
Carried out the catalyst preparation, characterization, and catalytic tests, and prepared the draft manuscript: Zhang B
Performed part of the catalyst characterization: Chu Z, Zhang J, Wu Z
Performed the TEM characterization: Yang D, Wu H
Planned the study, analyzed the data and wrote the manuscript: Shi J, Jiang Z
Availability of data and materials
Not applicable.
Financial support and sponsorship
This work was supported by the National Key R&D Program of China (2022YFC2105902), the National Key R&D Program of China (2021YFC2102300), the National Natural Science Funds of China (22122809), Open Funding Project of the State Key Laboratory of Biochemical Engineering (2020KF-06), and Tianjin Synthetic Biotechnology Innovation Capacity Improvement Project (TSBICIP-KJGG-003) for financial support.
Conflicts of interest
All authors declared that there are no conflicts of interest.
Ethical approval and consent to participate
Not applicable.
Consent for publication
Not applicable.
Copyright
© The Author(s) 2023.
Supplementary Materials
REFERENCES
1. Yaashikaa P, Senthil Kumar P, Varjani SJ, Saravanan A. A review on photochemical, biochemical and electrochemical transformation of CO2 into value-added products. J CO2 Util 2019;33:131-47.
2. Zhao T, Feng G, Chen W, et al. Artificial bioconversion of carbon dioxide. Chinese J Catal 2019;40:1421-37.
3. Markewitz P, Kuckshinrichs W, Leitner W, et al. Worldwide innovations in the development of carbon capture technologies and the utilization of CO2. Energy Environ Sci 2012;5:7281.
4. Hermida-Carrera C, Kapralov MV, Galmés J. Rubisco catalytic properties and temperature response in crops. Plant Physiol 2016;171:2549-61.
5. Cummins PL, Kannappan B, Gready JE. Directions for optimization of photosynthetic carbon fixation: RuBisCo’s efficiency may not be so constrained after all. Front Plant Sci 2018;9:183.
6. Itakura AK, Chan KX, Atkinson N, et al. A Rubisco-binding protein is required for normal pyrenoid number and starch sheath morphology in Chlamydomonas reinhardtii. Proc Natl Acad Sci USA 2019;116:18445-54.
7. Valegård K, Andralojc PJ, Haslam RP, et al. Structural and functional analyses of Rubisco from arctic diatom species reveal unusual posttranslational modifications. J Biol Chem 2018;293:13033-43.
8. Lindskog S, Coleman JE. The catalytic mechanism of carbonic anhydrase. Proc Natl Acad Sci USA 1973;70:2505-8.
9. Cao S, Yue D, Li X, et al. Novel nano-/micro-biocatalyst: soybean epoxide hydrolase immobilized on UiO-66-NH2 MOF for efficient biosynthesis of enantiopure (R)-1, 2-octanediol in deep eutectic solvents. ACS Sustain Chem Eng 2016;4:3586-95.
10. Cao S, Xu P, Ma Y, et al. Recent advances in immobilized enzymes on nanocarriers. Chinese J Catal 2016;37:1814-23.
11. Cao S, Xu H, Lai L, et al. Magnetic ZIF-8/cellulose/Fe3O4 nanocomposite: preparation, characterization, and enzyme immobilization. Bioresour Bioprocess 2017;4:1-7.
12. Alizadeh N, Salimi A, Hallaj R, Fathi F, Soleimani F. Ni-hemin metal-organic framework with highly efficient peroxidase catalytic activity: toward colorimetric cancer cell detection and targeted therapeutics. J Nanobiotechnology 2018;16:93.
13. Drout RJ, Robison L, Farha OK. Catalytic applications of enzymes encapsulated in metal-organic frameworks. Coord Chem Rev 2019;381:151-60.
14. Wu X, Hou M, Ge J. Metal-organic frameworks and inorganic nanoflowers: a type of emerging inorganic crystal nanocarrier for enzyme immobilization. Catal Sci Technol 2015;5:5077-85.
15. Doonan C, Riccò R, Liang K, Bradshaw D, Falcaro P. Metal-organic frameworks at the biointerface: synthetic strategies and applications. Acc Chem Res 2017;50:1423-32.
16. Riccò R, Liang W, Li S, et al. Metal-organic frameworks for cell and virus biology: a perspective. ACS Nano 2018;12:13-23.
17. Du Y, Gao J, Zhou L, et al. MOF-based nanotubes to hollow nanospheres through protein-induced soft-templating pathways. Adv Sci (Weinh) 2019;6:1801684.
18. Sun Q, Fu CW, Aguila B, et al. Pore environment control and enhanced performance of enzymes infiltrated in covalent organic frameworks. J Am Chem Soc 2018;140:984-92.
19. Serre C, Kitagawa S, Dietzel PD. Introduction to special issue: metal organic frameworks. Microporous Mesoporous Mater 2012;157:1-2.
20. Furukawa H, Cordova KE, O'Keeffe M, Yaghi OM. The chemistry and applications of metal-organic frameworks. Science 2013;341:1230444.
21. Eum K, Jayachandrababu KC, Rashidi F, et al. Highly tunable molecular sieving and adsorption properties of mixed-linker zeolitic imidazolate frameworks. J Am Chem Soc 2015;137:4191-7.
22. Zhang H, Hou J, Hu Y, et al. Ultrafast selective transport of alkali metal ions in metal organic frameworks with subnanometer pores. Sci Adv 2018;4:eaaq0066.
23. Luo J, Wang J, Zhang J, Lai S, Zhong D. Hydrogen-bonded organic frameworks: design, structures and potential applications. CrystEngComm 2018;20:5884-98.
24. Lin RB, He Y, Li P, Wang H, Zhou W, Chen B. Multifunctional porous hydrogen-bonded organic framework materials. Chem Soc Rev 2019;48:1362-89.
25. Hisaki I, Xin C, Takahashi K, Nakamura T. Designing hydrogen-bonded organic frameworks (HOFs) with permanent porosity. Angew Chem Int Ed Engl 2019;58:11160-70.
26. Luzuriaga MA, Benjamin CE, Gaertner MW, et al. ZIF-8 degrades in cell media, serum, and some-but not all-common laboratory buffers. Supramol Chem 2019;31:485-90.
27. Velásquez-hernández MDJ, Ricco R, Carraro F, et al. Degradation of ZIF-8 in phosphate buffered saline media. CrystEngComm 2019;21:4538-44.
28. Luzuriaga MA, Welch RP, Dharmarwardana M, et al. Enhanced Stability and controlled delivery of MOF-encapsulated vaccines and their immunogenic response in vivo. ACS Appl Mater Interf 2019;11:9740-6.
29. Sun CY, Qin C, Wang XL, et al. Zeolitic Imidazolate framework-8 as efficient pH-sensitive drug delivery vehicle. Dalton Trans 2012;41:6906-9.
30. Persico F, Wuest JD. Use of hydrogen bonds to control molecular aggregation. Behavior of a self-complementary dipyridone designed to self-replicate. J Org Chem 1993;58:95-9.
31. Russell VA, Evans CC, Li W, Ward MD. Nanoporous molecular sandwiches: pillared two-dimensional hydrogen-bonded networks with adjustable porosity. Science 1997;276:575-9.
32. Tamames-Tabar C, Cunha D, Imbuluzqueta E, et al. Cytotoxicity of nanoscaled metal-organic frameworks. J Mater Chem B 2014;2:262-71.
33. Grall R, Hidalgo T, Delic J, Garcia-Marquez A, Chevillard S, Horcajada P. In vitro biocompatibility of mesoporous metal (III; Fe, Al, Cr) trimesate MOF nanocarriers. J Mater Chem B 2015;3:8279-92.
34. Tang Z, Li X, Tong L, et al. A biocatalytic cascade in an ultrastable mesoporous hydrogen-bonded organic framework for point-of-care biosensing. Angew Chem Int Ed Engl 2021;60:23608-13.
35. Bao Z, Xie D, Chang G, et al. Fine tuning and specific binding sites with a porous hydrogen-bonded metal-complex framework for gas selective separations. J Am Chem Soc 2018;140:4596-603.
36. Tang J, Liu J, Zheng Q, et al. In-situ encapsulation of protein into nanoscale hydrogen-bonded organic frameworks for intracellular biocatalysis. Angew Chem Int Ed Engl 2021;60:22315-21.
37. Liang W, Carraro F, Solomon MB, et al. Enzyme encapsulation in a porous hydrogen-bonded organic framework. J Am Chem Soc 2019;141:14298-305.
Cite This Article
Export citation file: BibTeX | RIS
OAE Style
Zhang B, Shi J, Chu Z, Zhang J, Wu Z, Yang D, Wu H, Jiang Z. Lysine-modulated synthesis of enzyme-embedded hydrogen-bonded organic frameworks for efficient carbon dioxide fixation. Chem Synth 2023;3:5. http://dx.doi.org/10.20517/cs.2022.28
AMA Style
Zhang B, Shi J, Chu Z, Zhang J, Wu Z, Yang D, Wu H, Jiang Z. Lysine-modulated synthesis of enzyme-embedded hydrogen-bonded organic frameworks for efficient carbon dioxide fixation. Chemical Synthesis. 2023; 3(1): 5. http://dx.doi.org/10.20517/cs.2022.28
Chicago/Turabian Style
Zhang, Boyu, Jiafu Shi, Ziyi Chu, Jiaxu Zhang, Zhenhua Wu, Dong Yang, Hong Wu, Zhongyi Jiang. 2023. "Lysine-modulated synthesis of enzyme-embedded hydrogen-bonded organic frameworks for efficient carbon dioxide fixation" Chemical Synthesis. 3, no.1: 5. http://dx.doi.org/10.20517/cs.2022.28
ACS Style
Zhang, B.; Shi J.; Chu Z.; Zhang J.; Wu Z.; Yang D.; Wu H.; Jiang Z. Lysine-modulated synthesis of enzyme-embedded hydrogen-bonded organic frameworks for efficient carbon dioxide fixation. Chem. Synth. 2023, 3, 5. http://dx.doi.org/10.20517/cs.2022.28
About This Article
Copyright
Author Biographies
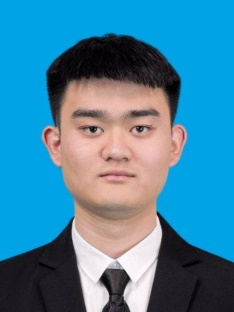
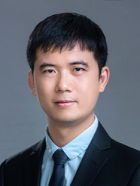
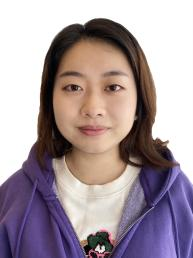
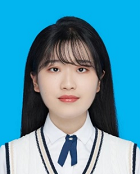
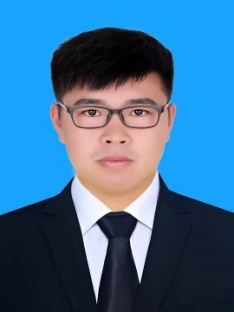
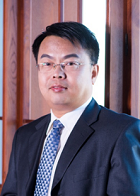
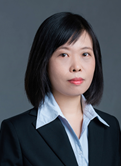
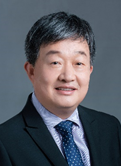
Data & Comments
Data

Comments
Comments must be written in English. Spam, offensive content, impersonation, and private information will not be permitted. If any comment is reported and identified as inappropriate content by OAE staff, the comment will be removed without notice. If you have any queries or need any help, please contact us at support@oaepublish.com.